|
New post on The Stem Cellar |
|
|
“As a year like no other ends, we begin to look forward to 2021 and the possibilities ahead. At the start of 2020, we were focused on the Phase II clinical trial using the BCG vaccine in adults with established type 1 diabetes. We were also planning for the Expanded Access program and a long anticipated pediatric clinical trial. All of these steps are underway and our commitments to type 1 diabetes will never waiver, but COVID-19 has created a new challenge and amazing opportunities for the type 1 diabetic community.
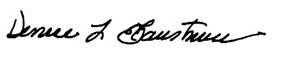
Origin of the coronavirus SARS-CoV-2 by Amy Rogers
“I am suddenly seeing a lot more chatter and innuendo suggesting, or flat-out accusing, that SARS-CoV-2 came from a Chinese laboratory.
“Before you spread these rumors, consider these things.
“First, keep clear in your mind the difference between two different accusations:
- That China (or insert your favorite enemy here) created or genetically engineered this new virus for use as a bioweapon
- That a laboratory studying coronaviruses had an accident or security breach resulting in the escape of one of those viruses into the community
“#1 should leave evidence in the genetic sequence of the virus. As I blogged previously, experts say there is no such evidence in the genome of SARS-CoV-2. So as far as I am concerned, #1 is false. People say it, share it, believe it for the same reason all conspiracy theories find traction: people are afraid, and pinning blame on powerful dark forces gives a sense of control.
“So let’s talk about #2.
“Fact: there is a biosafety level 4 (highest level) virology research lab in Wuhan. Fact: this facility is well-known for its research on coronaviruses. Fact: some of the research there involves going into caves and other places where wild bats live, collecting bat guano, and studying viruses found in bats. The lab would definitely have both live and frozen specimens of a variety of coronaviruses.
“BSL-4 laboratories are explicitly designed to isolate the most dangerous microorganisms known—agents that are deadly, and have no vaccine or treatment. Is it possible that an accident could occur, and a worker could be exposed? Of course. Is it possible that an employee intentionally breached protocol for some reason, and brought a virus into the community? Of course it is possible.
“But is there evidence of this, or mere coincidence? For myself, I don’t spread rumors without evidence.
“Apparently not everyone is like me. Here’s what happened this week.
“On Tuesday, American’s highest-ranking military officer, Gen. Mark Milley, chairman of the Joint Chiefs of Staff, weighed in on the speculation that the origin of SARS-CoV-2 is not natural. The general’s exact quote was,
“At this point, it’s inconclusive although the weight of evidence seems to indicate natural {origin}.”
“Two major news organizations both covered the story. In the body of their articles, both reported the same basic information. But they chose very different emphases.
Headline A: U.S. Military Says Coronavirus Likely Occurred Naturally but Not Certain
Headline B: Sources believe coronavirus originated in Wuhan lab as part of China’s efforts to compete with US
“I would add that in article B, the “sources” who “believe” the virus came from the Wuhan lab are unnamed.
“I’m not interested in the agenda behind this spin. I’m interested in the scientific facts of how this new zoonotic (animal) virus found its way from bats into the people of Wuhan. There are countless plausible ways.
“An accident at the Wuhan Institute of Virology is one possibility. Evidence is lacking.
“An intermediate animal host such as pangolin at the Huanan Seafood Wholesale Market is another. Evidence is admittedly weak. Although the first recognized COVID-19 disease cluster was centered on the market, the first human cases (probably in November, as first official case was Dec. 1) might have brought the virus into the market rather than the other way around.
“The list of other possible routes is nearly infinite. Maybe it was some poor kid playing in the dirt outside a bat cave. A wealthy Wuhan citizen doing something weird with a bat for traditional Chinese medicine. Wildlife or a pig slaughtered in somebody’s back yard.
“The key point is, we may never know for sure. Uncertainty makes us uncomfortable, but don’t let that drive you to a false certainty. In the absence of proof, carefully examine your own worldview, what biases you have, that encourage you to “believe” one possible truth over another.
“In the end, I’d like to emphasize that we have long expected a virus like this to emerge naturally. (Even Hollywood knew: see the movie Contagion.) We have previous examples: see SARS-CoV-1, and MERS, zoonotic coronaviruses that jumped into humans naturally. And I can guarantee that another virus, and another, and another, will make this leap on their own without any laboratories or conspiracies in the future.
“(If you’d like to learn more about the science of how a virus jumps species, see my February treatise on the coronavirus.)
“Amy Rogers, MD, PhD, is a scientist, novelist, journalist, and educator. Learn more about Amy’s science thriller novels, or download a free ebook on the scientific backstory of SARS-CoV-2 and emerging infections, at AmyRogers.com.
“Did you know that my third science thriller novel, The Han Agent, is about a viral bioweapon and China?”
…”Stuck at home with no books to read? Here are 6 solutions.”
“Different parts of the U.S. are experiencing different levels of shutdown, but one thing is universal: Books make staying at home a much better experience.
“But what if your bedside stack of reading material is down to its last book? And now you can’t reach the place you normally get books or spend time with the friend with whom you trade books?
“Don’t despair, dear readers. Here are five ways to get books instantly through Amazon or Audible, all of which require only a mobile phone or tablet.
“Plus I have one bonus suggestion at the very end that will take quite a bit longer than “instantly” but might change your life….
“Prime Reading. Prime Reading offers thousands of e-books and magazines that are free to download for Amazon Prime members. Browse through the books available in Prime Reading, download the Kindle app to your phone or tablet, and then download the books you want to read. The choices are constantly changing, but at the moment a few highlights include Margaret Atwood’s The Handmaid’s Tale, K.F. Breene’s Sin & Chocolate, and a cornucopia of Dean Koontz suspense novels. There are e-books for kids who are comfortable reading on phone or tablets, including Rick Riordan’s The Lost Hero and the Frozen junior novel.
“Kindle Unlimited. Amazon’s Kindle Unlimited offers its members unlimited access to download more than a million books and magazines. Right now Kindle Unlimited is offering a 30-day free trial for its subscription service. There are so many titles available in Kindle Unlimited, how to pick what to read first? You can start with our recent articles on Women’s History Month and World Storytelling Day that feature books in Kindle Unlimited. Or when you Search on Amazon in the Kindle store, make sure you check the option for “Kindle Unlimited Eligible.” (On your web browser, you will find the checkbox on the left side of the screen. On your Amazon app, you will find the checkbox as an option under “Filters.” The Kindle Unlimited Eligible option only shows up when searching titles within the Kindle store, please note.)
“There are many, many other ways to find Kindle books you’ll love. If you have a careful eye on your bank account, check out Kindle Daily Deals, which spotlight great reads on sale. And Amazon First Reads offers one Kindle book per month for only $1.99 (or for free if you’re an Amazon Prime member) from a selection of eight titles.
“Audible. The master in the audiobooks arena (and also an Amazon company), Audible very likely has the audiobook version of whatever book you want to read. And audiobooks can resonate in ways that reading words do not. For instance, I cannot think of Jim Butcher’s Dresden Files novels without remembering James Marsters’s growly voice, or of the Harry Potter series without hearing award-winning narrator Jim Dale. While you can purchase audiobooks a la carte, a monthly Audible membership also includes two of their amazing Audible Originals. (I recently painted my bathroom while listening to the Audible Original A Grown-Up Guide to Dinosaurs, and it made the hours fly by.) But wait: There’s more! Audible just launched Audible Stories, a collection of free audiobooks that are aimed at kids from toddlers to teens but also includes classics for readers of all ages. Download the Audible app to get started.
“Here’s the not-instantaneous solution I mentioned earlier in this article: Write your own book. Or poem. Or short story. Or graphic novel. Just start writing and see where it takes you. Most authors have first or second or third careers before they start writing, so don’t think you need a master’s degree in literature or have to go on a writers’ retreat to be worthy of putting a pen to paper (or fingers to the keyboard). We all have stories inside of us. Take this opportunity to let them out.
“This article was originally published in slightly different form on March 23, 2020.”
As usual, Just sharin’. #buckroth
“8 Reasons Why Insulin is so Outrageously Expensive”
20 Jan 2019, 6:08 p.m. in #insulin4all USA by T1International
https://www.t1international.com/blog/2019/01/20/why-insulin-so-expensive/
“Why does insulin cost so much to patients in the USA and around the world? Why is insulin, a widely sold drug of which most forms are now off-patent, so incredibly expensive? These are simple questions, but ones with a number of complicated answers. This post will break some of those answers down and point you in the direction further reading if you want to dive deeper.
“1. Only 3 Companies Control 90% of the Global Insulin Market
“The ‘big three’ insulin producers – Eli Lilly, Novo Nordisk and Sanofi – dominate more than 90% of the world insulin market by value. Often only one of these companies supplies insulin in a country, which means they more or less hold a monopoly there and can set prices as they wish. In some countries, notably China and India, there are domestic insulin companies that can help drive down the price. This means we need more companies in markets like the USA to help bring prices down. We’ll touch on that a bit further down the list.
“2. No Generic Insulin
“When it comes to the question of generic insulin, we are faced with another complicated issue. Insulin is a therapeutic biological product (or ‘biologic’), rather than a chemically synthesized molecule. This means it cannot be made as generic in the same way as other drugs. Creating what is called a biosimilar is a lot more complicated and expensive than just duplicating a chemical molecule. There is little market incentive to produce biosimiliars because it costs nearly as much as making new drug, and companies must go through all the approval stages and trials that a new drug is required to go through. Not to mention, current biosimilar insulins on the market – primarily produced by the ‘big three’ – have only reduced the price by about 10-15%. For more on biosimilars and the 2018 FDA announcement read this and this.
“3. Pay-for-Delay Schemes & Lawsuits
“A ‘Pay for delay’ agreement is a patent dispute settlement in which a generic (in the case of insulin, a biosimilar) manufacturer acknowledges the original patent of a pharmaceutical company and agrees to refrain from marketing its product for a specific period of time. In return, the company receives a payment from the patent-holder. This means it is actually legal for one insulin producer to pay another one not to enter the market. A few years ago the company Merck announced plans to sell a biosimilar version of Sanofi’s Lantus. Sanofi sued, and eventually Merck announced that it was no longer pursuing it’s biosimilar, presumably due to payments from Sanofi to stay away. If Pay for delay schemes don’t work, the ‘big three’ can still sue other players, prolonging processes and pushing players out of the market because of legal fees and time-wasting. All of these are win-wins for companies, and lose-lose for patients.
“4. Patents
“Why aren’t we seeing more companies making insulin? There are many reasons for this, but patent evergreening is a big one. Patents give a person or organization a monopoly on a particular invention for a specific period of time. In the USA, it is generally 20 years. Humalog, Lantus and other previous generation insulins are now off patent, as are even older animal based insulins. So what’s going on? Pharmaceutical companies take advantage of loopholes in the U.S. patent system to build thickets of patents around their drugs which will make them last much longer (evergreening). This prevents competition and can keep prices high for decades. Our friends at I-MAK recently showed that Sanofi, the maker of Lantus, is no exception. Sanofi has filed 74 patent applications on Lantus alone, that means Sanofi has created the potential for a competition-free monopoly for 37 years.
“5. Politics
“Companies are not in the habit of throwing money away, and they are not in the habit of staying out of politics. Eli Lilly, Novo Nordisk, and Sanofi collectively rake in several billions of dollars in profits. That’s not millions, but billions – with a B. We know they spend millions on marketing, but they also spend millions on lobbying politicians and donating to our decision-makers so that they keep quiet about price gouging. Check if your representatives receive contributions from one of the ‘big three’ insulin manufacturers or any pharmaceutical company. Chances are, they do. Not to mention, the revolving door between pharma companies and US Government positions. Our current secretary of Health and Human Services was previously an Eli Lilly executive. Obviously, his interests are not with people, but with power. This is why independent patient voices are so important.
“6. Price Fixing
“These Business Insider graphs pretty much say it all.
Several lawsuits alleging some form price-fixing are currently in the works. You can read more here and here.
“7. Pharma Marketing Schemes
“Physicians in the United States and some other countries are allowed to collect fees from pharmaceutical companies for talks, advice, and more. Supposedly, these are to compensate physicians for their expertise and time. However, they can create loyalty to a company and may influence prescribing habits – a belief shared by some pharmaceutical salespeople. In some countries like India, physicians are allowed to sell and profit off insulin directly through patients, or through pharmacies they themselves own, cutting out middlemen and the retail pharmacies. Thus, they lose the incentive to find the lowest price insulin for their patients. Insulin companies also focus on ‘insulin-starts’, or the insulin the physician diagnosing patients begins with. As patients are reluctant to change, a number of marketing and financial incentives are employed to influence this decision.
“8. Payment for Influence (or Silence)
“Many major key opinion leaders, influencers, and patient advocacy organizations take pharma cash. For example, the two biggest diabetes organizations – The American Diabetes Association and The Juvenile Diabetes Research Foundation – have accepted huge sums from insulin manufacturers. Other groups were actually created by money from the ‘big three’, like the World Diabetes Foundation which is funded by Novo Nordisk, and other supposed advocacy groups that are actually doing pharma’s bidding, or at least are highly influenced by them. If this issue is important to you, check the funders of an organization you want to support, and if it’s not transparent, you can ask if they take industry money.
“What Can be Done?
“Patients are speaking out about these issues all around the world. In the USA where prices have skyrocketed especially, T1International Chapters are being formed where patient advocates are educating and pushing for policy change. You can learn more about Chapter here and find more information and action related to the insulin price crisis here. Whatever you do, wherever you are – don’t stay silent.”
For the complete informational article, please click on this link: https://www.t1international.com/blog/2019/01/20/why-insulin-so-expensive/. Thank you!
Gosh, what a mess! I am T1D. I know the pains. Just sharin,’ once again. #buckroth
https://diabetesresearchconnection.org/news-2/
SAVE THE DATE
3rd Annual Dance for Diabetes
UNDERTH THE SEA
The Best Rooftop Party of the Year!
September 19, 2020
6 PM – 11 PM
1400 Maiden Lane, [Del Mar Plaza]
Del Mar, California 92014
Benefiting Diabetes Research Connection
info@DiabetesResearchConnection
(844) 484-3372
https://diabetesresearchconnection.org/news-2/
Maybe I will see you there. Look for me! #buckroth